at ginkgo, it’s my job to coax organisms into performing new tricks—producing compounds and proteins they would never make of their own accord. In today’s experiment, I am trying to convince yeast cells to accept DNA spliced together from three different plants. Unfortunately, when I get to work, I find that my cells have been gorging on last night’s nutrient broth and are on the verge of passing out. I see them under the microscope, plump, round, hung over. Annoyed, I take a few of them, dilute them into fresh nutrient broth and wait. I need them to be on their toes for the task at hand. While they wake up, I retrieve a small vial from the freezer and place it on a bed of crushed ice. It contains invisible strands of precious plant DNA, which I’ve trimmed with molecular scissors. The yeast will soon take up these strands of DNA and, if its series of A’s, T’s, C’s, and G’s are in the right order, will start interpreting it and producing plant proteins. These cells will harbor snippets from the plants’ essence — a message from cousins it last met billions of years ago. What is this new thing I’ve constructed, and what is my relationship to it? It will still be yeast, but it will also be something else — a new living being the world has never seen before. And I’ll be one of the people to determine whether it’s useful and should be propagated further. However, while I am the author of this experiment, I am at best the co-author of the novel organism that eventually emerges. After all, I have access to the plants’ DNA thanks to an intergenerational and international supply chain: countless millennia of natural selection, dozens of generations of humans and nonhumans who directed their evolution and kept them alive, several generations of scientists who learned to synthesize their essence, not to mention the team at Ginkgo that prepared them for me. Who owns this crystallization of our heritage, this tiny new branch of life? A tough question.
New Protocols
The ownership of biological resources has always been hard to delineate. Unlike fossil fuels or minerals, biology is self-regenerating, and most organisms can usually be cultivated any number of places outside of their place of origin. On a small scale, gardeners and bakers are comfortable with this: Every time they split off a cutting or pinch off a bolus of sourdough starter for a friend, they face the reality that they are only custodians of the plants and microbes they cultivate and enjoy.
But trading biological resources takes on a very different tenor when it comes to corporate ownership. In 1997, for example, Texas-based food corporation RiceTec succeeded in convincing the U.S. Patent Office to issue a broad patent covering their trademarked hybrid basmati rice, giving the company de facto exclusive rights to basmati hybrids grown or sold anywhere in the United States. The name of their product — which was created using traditional methods of plant hybridization — was included in the patent (“Basmati Rice Lines and Grains”), suggesting that RiceTec had some legal claim on the word “basmati” as well.
An American company was claiming a cultural heritage from across the globe. Named for its prized aroma (from the Sanskrit vas — fragrant — and mayup — ingrained from the beginning), basmati has been a celebrated part of Indian and Pakistani history, culture, and economy for centuries. What’s more, RiceTec’s patent posed an immediate threat to the livelihoods of rice farmers in India, not to mention the country’s economy, which at the time was estimated to be producing and exporting over 600,000 tonnes of basmati rice every year. Food activists staged major protests. India and Pakistan, in a rare moment of solidarity, appealed together to the World Trade Organization. It took five years and further legal measures by the Indian government for the patent claims to be reduced to the specific hybrid plant RiceTec had bred, and for the Texas company to content itself with the sales of their trademark “Texmati” in the United States.
This was just one high-profile example of a corporation reaping benefits from resources originally cultivated by traditional growers. Companies and institutions from affluent countries have long been harvesting valuable biological ingredients from less affluent countries. This extraction often occurs without returning any benefit or meaningful acknowledgment of the peoples’ development and stewardship of these biological resources.
In an attempt to address this known driver of inequality, more than 127 nations — including many in Europe, Asia, Africa, South America, Australia, but, notably, not the U.S. — signed the 2010 Nagoya Protocol. An expansion of the 1992 Convention on Biological Diversity, Nagoya establishes internationally agreed terms for access and benefit-sharing of the genetics of biodiversity. Rather than restricting the trade of genetic resources, the protocol identifies how nations can exercise sovereignty in defining access to genetic resources and be compensated for allowing this access by benefit-sharing agreements. Nations who fostered biodiversity now have a way to stake a claim to the output of corporations or other institutions that seek to profit from it. For a time, at least, Nagoya seemed to have brokered a fragile peace.
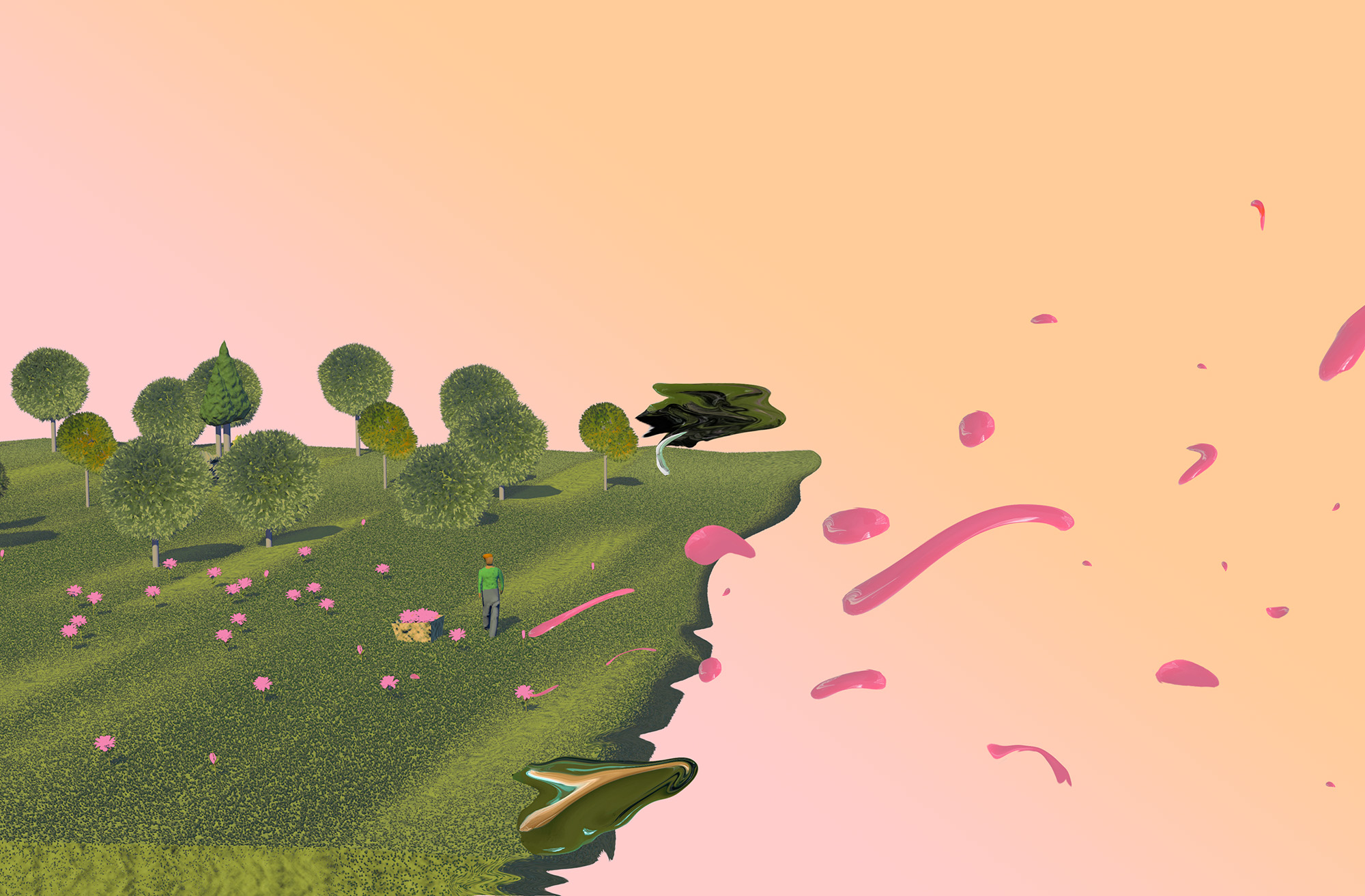
Uncoupled from Natural Selection
Recent advances in biotechnology, however, have made things a lot more complicated. When I transform my yeast with plant DNA, I haven’t extracted that genetic material directly from an actual plant. Instead, I have chemically synthesized it from sequences that are stored in publicly available databases . My designer DNA has been shaped for optimal function in yeast. In a single research campaign, I may synthesize hundreds or even thousands of bits of DNA from plants and microbes from all over the world that I have never physically encountered.
Biochemist H. Gobind Khorana was one of many scientists who led us down this path. Working at the University of Wisconsin in the 1960s, he studied how cells interpreted genetic code. In an elegant series of experiments, he fed a repeating sequence of genetic material to cellular machinery and analyzed the output. Before long, he had elucidated how the sequences of A’s, T’s, C’s, and G’s that comprise DNA get translated into proteins in the cell — the core of all life. This Nobel Prize-winning work allowed biologists to identify what proteins are encoded in the genome of organisms, and would later enable the world to develop mRNA vaccines against SARS-CoV-2.
Crucially for the development of synthetic biology, Khorana’s experiment chemically synthesized chains of nucleic acid, the building blocks of a cell’s DNA and RNA, unleashing our ability to re-engineer living cells and natural systems.
Khorana’s DNA writing tools found their natural counterpart a decade later, when Fredrick Sanger set out to read these mysterious sequences. In 1975, working with Alan Coulson, he developed a method that took advantage of a cell’s native ability to synthesize DNA, using radioactively labeled nucleotides instead of the ones naturally found in the cell. These radioactive pieces could be analyzed to reveal the make-up of a specific snippet of DNA — opening it up for reading. This new method revolutionized biology: Scientists could now peer inside of cells and understand what basic building blocks drive their biology. Sanger shared half of the 1980 Nobel Prize in Chemistry with Walter Gilbert for his efforts; in a sign of things to come, the other half of the prize was awarded to Paul Berg for splicing two pieces of DNA together — another core technology for engineering organisms.
These breakthroughs yielded a powerful set of biological tools. In 1982, the National Center for Biotechnology Information in the U.S. started a collection of all known DNA sequences. In its early years, this database could easily fit in a single binder; later, a bookshelf. By most recent estimates, the number of sequences deposited in this database is growing exponentially, doubling roughly every 18 months. As of this April, the database contained over 2 billion protein sequences, a miniature of the natural world.
Reading and writing DNA, which allows scientists to treat biological entities (plants, animals, microbes) like computers interpreting code, is the core of synthetic biology. Frances Arnold won the 2018 Nobel Prize for the ability to direct the evolution of genes towards new functions; soon we may be able to generate DNA sequences never found in nature and predict which ones will work best to achieve a particular objective. Evolution is progressively being uncoupled from natural selection.
This could produce great innovation, wealth, and advancements in the health of humans and the environment. Rice enriched with genes that provide a boost in vitamin A production could reduce childhood mortality. Yeast that can convert sugar into high-grade ethanol could relieve energy crises and oil production dips. New ways of growing animal-free dairy and meat products — extracting genetic material that provides dairy and milk their signature flavors and textures, and expressing them in microbial hosts — could lessen the burden of factory farming and mitigate cruelty to farmed animals.
How do we define biological property in this new world? A product of the 2000s, the Nagoya Protocol did not anticipate the rapid evolution of such approaches. The protocol focused on physical transfer of genetic material, rather than the digital sharing of information. Our previous frameworks for understanding ownership and sharing benefits are quickly becoming obsolete.
Culture and Biology
Synthetic biology challenges the Nagoya Protocol with a new ability to view DNA as an information stream fluidly changing and re-interpreted outside of its original context. It has added a new dimension to biological ownership and its impacts on cultural heritage. There is great promise in this elegant new technology, but without consideration, it could also just become the latest tool to commodify and exploit natural wealth.
Vanilla is a good example of this complicated long-running cycle. The orchid that originally elicited this wonderful fragrance is tied to brutal military and economic campaigns waged by different ethnicities and peoples. By current reckoning, the bean was first cultivated by the Totonac peoples in Mesoamerica, who introduced it to the Aztecs during their war in the 15th century. Hernán Cortés’ conquests for the Spanish Empire in the Americas introduced the flavoring to Europe, which developed a growing market for it. In an economic en passant, French colonists transported the orchid from the Americas across the Atlantic Ocean to Réunion in the Indian Ocean. In the 19th century, pollination innovations created a boom of East African vanilla in North America, which shifted prominence away from Mexican vanilla producers to those that of the Indian Ocean, where it remains an important industry. One final twist came from organic chemistry in the twentieth century: Synthetic vanilla or vanillin, derived from fossil fuel byproducts or plant oils — now dominates the vanilla market.
Synthetic biology is the latest spin-off in this lineage. In 2014, the Swiss company Evolva announced the ability to brew vanillin from yeast by “reading” the DNA segments necessary for producing a vanilla fragrance from the orchid — “writing” that DNA, borrowing sequences from a range of bacteria, fungi, plants, and even animal sources, and then introducing it to yeast. Evolva has patented its new GMO. It now licenses it to its partners who can produce vanillin by the liter in fermentation vessels instead of the traditional, labor-intensive, and ecologically costly method of harvesting and processing vanilla beans. Advocacy groups have argued that this biological innovation in producing vanilla could undercut a livelihood that has developed in the economies that currently depend on the vanilla trade, particularly in Madagascar.
Evolva’s iteration of vanilla may be disruptive for many more reasons. Unlike chemically-synthesized vanillin, its biological iteration is no longer tied to the price or environmental impact of fossil fuels and can be produced on-demand in fermentation facilities around the world. What’s more, Evolva’s yeast-derived vanilla opens up a new market for vanilla flavor that is biologically derived. The company profits from readily available public resources — DNA sequences, biological technologies — that allows a legal claim of ownership over new organisms that produce the fragrance.
The economic journey from across the Atlantic to the Indian Ocean, from fossil fuel plants to fermentation tanks, has allowed me and countless others to know vanilla as part of our lives and personal histories. Ideally, it would be through an understanding of this history that we would determine which vanilla product we prefer: beans from Mexico or Madagascar; the synthetic vanillin from chemistry or biology.
The tools of the last 50 years that have built synthetic biology are poised to disrupt any number of industries: fragrances, dyes, fabrics, and even fuels that have their own complicated, long histories. Physical ownership of organisms and natural resources is no longer necessary for the production of commodities. The cellular information encoded in DNA can travel separately, estranged from its biological origins. Biology is transcending national boundaries and peoples. This new freedom requires some deep consideration. Synthetic biology ushers in a world in which we need new regulations surrounding genetic ownership beyond the Nagoya Protocol. It compels us to find new ways to share the economic benefits of biological resources — new ways that acknowledge their cultural importance and lineage — their status as something greater than commodities. This is not only a message of fairness. It is essential for understanding the meaning of the objects that surround us.
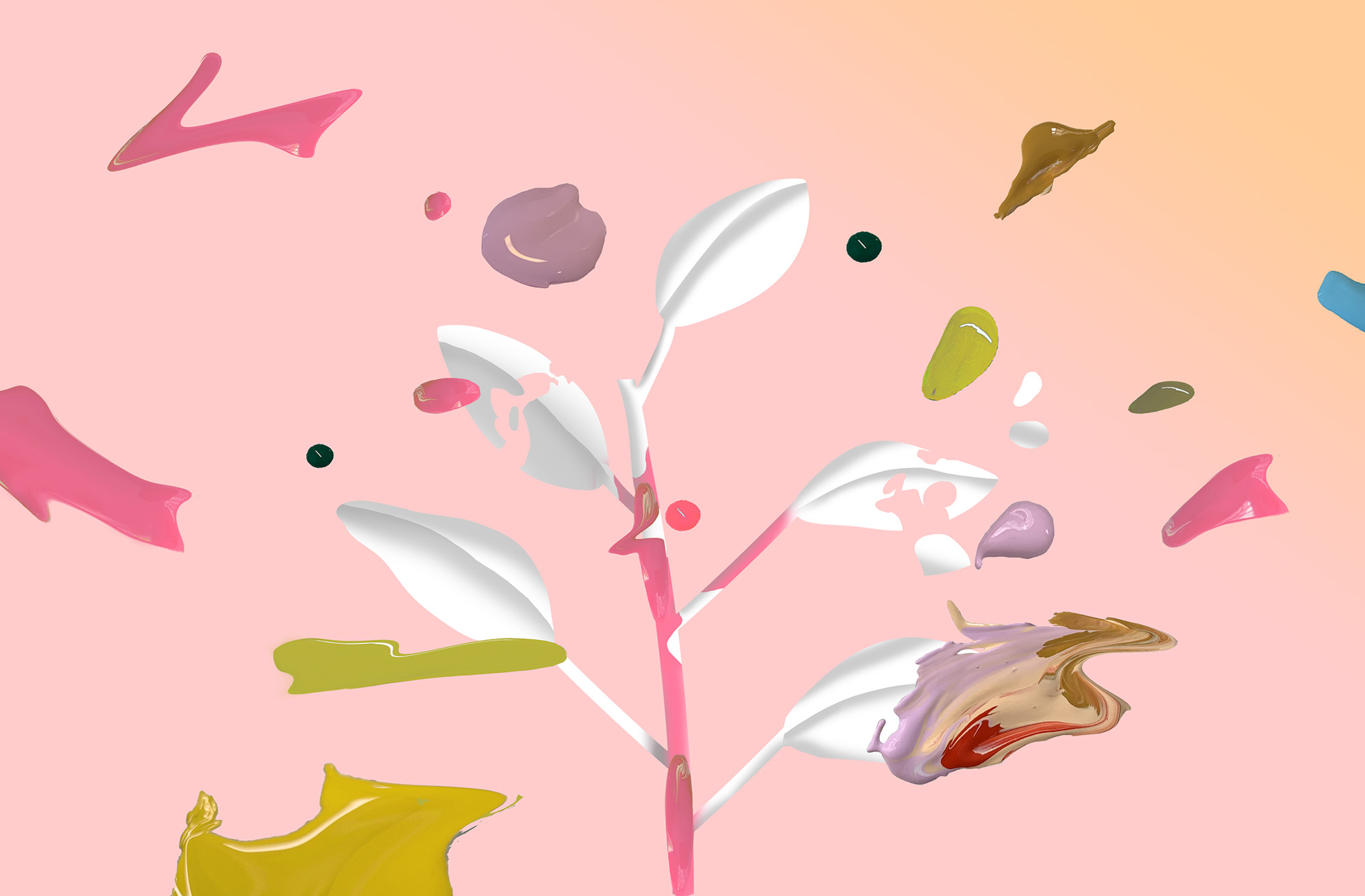
Zooming In
Sitting at my bench in Ginkgo Bioworks’ labs in Boston, I wonder about my role in this new, porous, biological world. My relationship with Ginkgo is uncomplicated: I provide my own experience and labor as a yeast geneticist and synthetic biologist and help Ginkgo grow. For this work, I am compensated and awarded equity in the company. Our fortunes are yoked — Ginkgo’s success is mine as well. This gives me a double stake in these plates and flasks that litter my bench.
My colleagues and I work with a menagerie of organisms — bacteria, fungi, even cells scraped and cultivated from animals — isolated from around the world, developed through centuries of careful observation and toil. The projects that come to us arrive from industries all over the globe that have been developed as a result of millennia of human knowledge passed through generations, drawing on eons of biological diversity in the making.
I find this work fascinating. Today, I am manipulating a new strain of yeast. I’m in love with this new strain. It grows at the cadence I expect it to and responds well to all the chemical queues I send its way. But it only does that because of the labor and knowledge developed by generations of unnamed brewers, vintners, and, later, scientists laboring in academic labs and industrial settings. The yeasts you encounter in the wild are never this well-behaved.
I’m keenly aware of the power of the technology in my hands — I am humbled by the new opportunities it presents — but I also know that it could exacerbate the already intolerable disparities in the world if access to it remains a relatively exclusive privilege. Who will have a say in this new frontier of human-driven evolution, and who will benefit from it? Hopefully, the answer will be: all of us.